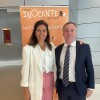
NOTICIAS, EVENTOS Y TEMAS DE ACTUALIDAD
La Fundación ALPE Acondroplasia ha sido una de las beneficiarias de las ayudas d... Leer más
¿Somos una sociedad decente? 17 de febrero de 2024, 17.00 -20.00 Sala Anfiteatro... Leer más
La necesidad de formación de las personas con Acondroplasia y otras Displasias E...
La labor de búsqueda de fondos por parte de la Fundación ALPE para poder financi... Leer más
Laura Garde, nutricionista de la Fundación ALPE, ha estudiado la acondroplasia d...
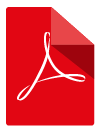
Documental colombiano dirigido por el Dr. Oscar Javier Cepeda Molina, sobre las...
El pasado 30 de noviembre de 2023 se presentó en el Parlamento Europeo el SD Ide... Leer más
BrideBio, la compañía biofarmacéutica de la que forma parte QED, ha emitido una...
El jueves 30 de noviembre de 2023 presentaremos en el Parlamento Europeo la hoja... Leer más
Sofía ha escrito un cuento. No solo lo ha escrito, sino que lo ha convertido en...
Ayer, día 25 de octubre, Día Internacional de las Displasias Esqueléticas que co...
Prometedores resultados de la fase 2 (ACcomplisH) del ensayo clínico sobre el Tr...
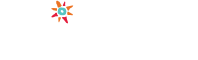
La Fundación ALPE Acondroplasia fue creada el 24 de enero de 2000 gracias al entusiasmo de varias personas, Carmen Alonso, Miguel López y la familia Press-Lewis, fundamentalmente. La familia Press-Lewis había fundado ProChon Biotech Ltd. en Tel-Aviv (Israel) para la búsqueda de una terapia para la acondroplasia. ProChon fue germen de avances científicos en la investigación de la acondroplasia que dan frutos cada vez más interesantes.
ENLACES
CONSULTAS MÉDICAS
Fundación ALPE Acondroplasia
Calle Conde Real Agrado, 2
33205 Gijón